Bioelectricity in Action: Guiding Development, Repair, and Regeneration
- Dr Luigi Vorluni
- Sep 2, 2024
- 5 min read
Updated: May 8
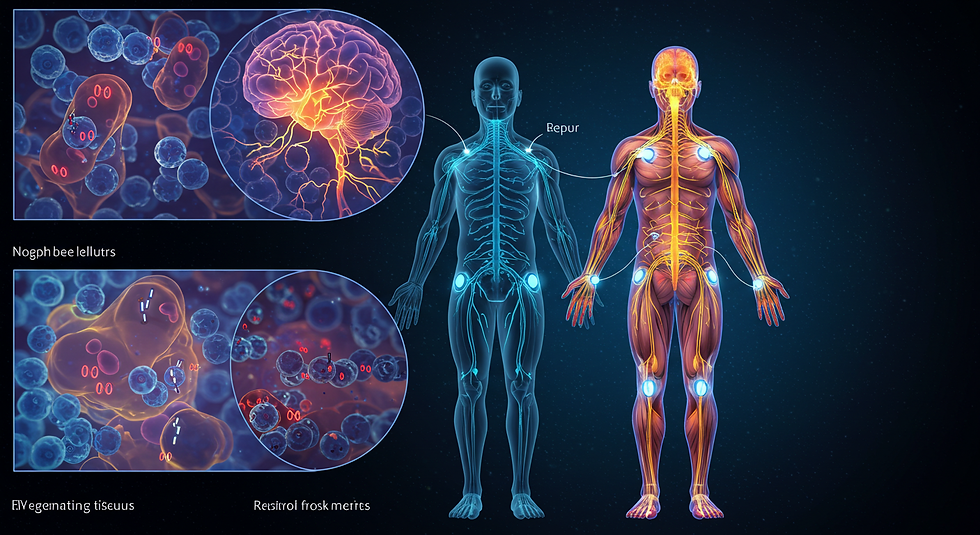
The Spark of Life: How Bioelectricity Shapes Healing, Growth, and Communication in the Body
From the firing of neurons in the brain to the healing of wounds and even the regeneration of limbs in some animals, bioelectricity plays a central role in life as we know it. This hidden electrical world, buzzing quietly within and between our cells, is becoming one of the most exciting frontiers in biology and medicine.
What Is Bioelectricity?
Bioelectricity is the flow of charged particles—ions like sodium, potassium, calcium, and chloride—across cell membranes. All living cells use this ionic current to function, but some, like nerve and muscle cells, are especially “excitable,” producing stronger, easily measurable signals like those seen in ECGs, EEGs or EMGs.
These electrical signals are not just a by-product of life; they are essential to it. From heartbeats to brain activity, motion, sensation, and even digestion—none of it would work without this continuous dance of electrical charge.
Healing and Regeneration: Guided by Bioelectrical Activity
When tissues are injured, bioelectric signals change immediately, guiding stem and specialised cells to the wound and kickstarting the healing process. This response is more than just a clean-up operation; in highly regenerative species like salamanders and flatworms, bioelectricity helps orchestrate the regrowth of entire limbs. Incredibly, scientists have shown that applying electric currents can even change the outcome of this process—reversing polarity in flatworms, for example, can make a tail grow where a head should have formed.
These findings suggest that bioelectricity acts like a “language” for cells, telling them where they are, what to become, and how to rebuild. And because this system is present in all animals—including humans—researchers are now exploring how it could be used to stimulate regeneration in tissues and organs that typically do not regrow.
A Hidden Geometry of Life
Studies have revealed that the body’s bioelectrical system follows a kind of spatial blueprint. The skin, for example, acts like a giant battery, with different body regions exhibiting unique electric potentials. Even at the cellular level, electrical polarity helps motor proteins navigate the complex interior of the cell, delivering cargo exactly where it’s needed.
In neurons, this polarity directs the flow of signals from dendrites (inputs) to axons (outputs), creating the one-way communication vital for brain function. This geometric organization extends across tissues, organs, and systems, linking the body's form and function to its internal electrical code.
Electricity in Medicine: The Future Is Now
Beyond understanding biology, bioelectricity has promising medical applications. In clinical settings, mild electrical stimulation is already being used to accelerate wound healing—especially in patients with chronic or slow-healing injuries. In the future, targeted bioelectric therapies may be able to prompt regeneration in human tissues that currently cannot regrow.
Still, much remains to be discovered. Regenerative medicine, which aims to restore lost or damaged tissues, is increasingly turning to bioelectricity for answers. But to translate lab discoveries into clinical treatments, scientists must continue to decode this subtle but powerful system—requiring collaboration across biology, physics, and engineering.
Bioelectricity: Understanding Life and the Future of Regenerative Medicine
As researchers delve deeper, bioelectricity is emerging as more than just a biological curiosity—it may be one of the fundamental forces shaping life, from the cellular level to entire organisms. This electrical network plays a role in guiding development, promoting healing, and maintaining the body's structure and function. It could hold the key to unlocking new approaches for treating disease, injury, and degeneration.
Could the precise application of electrical impulses one day help us regenerate damaged organs or regrow lost limbs? Science is getting closer to answering that question. As our molecular understanding of bioelectrical phenomena advances, it is opening new avenues for clinical applications in regenerative medicine.
References
Becker, R. O. (1967). The electrical control of growth processes. Medical Times, 95:657–669.
Levin, M. (2012). Morphogenetic fields in embryogenesis, regeneration, and cancer: non-local control of complex patterning. BioSystems, 109, 243–261.
McCaig, C. D., Rajnicek, A. M., Song, B., & Zhao, M. (2005). Controlling cell behavior electrically: current views and future potential. Physiological Reviews, 85(3), 943–978.
Nuccitelli, R. (2003). A role for endogenous electric fields in wound healing. Current Topics in Developmental Biology, 58, 1–26.
Zhao, M. (2009). Electrical fields in wound healing—an overriding signal that directs cell migration. Seminars in Cell & Developmental Biology, 20(6), 674–682.
Agata, K., & Inoue, T. (2012). Survey of the differences between regenerative and non-regenerative animals. Development Growth & Differentiation, 54(1), 143–152.
Borgens, R. B., Vanable, J. W., & Jaffe, L. F. (1977). Bioelectricity and regeneration: Large currents leave the stumps of regenerating newt limbs. Proceedings of the National Academy of Sciences, 74(10), 4528–4532.
McLaughlin, K. A., & Levin, M. (2018). Bioelectric signaling in regeneration: Mechanisms of ionic controls of growth and form. Developmental Biology, 433(2), 177–189.
Reddien, P. W., & Alvarado, A. S. (2004). Fundamentals of planarian regeneration. Annual Review of Cell and Developmental Biology, 20, 725–757.
Bessonov, N., Levin, M., Morozova, N., et al. (2015). Target morphology and cell memory: A model of regenerative pattern formation. Neural Regeneration Research, 10(12), 1901–1905.
Baker, L. L., Chambers, R., DeMuth, S. K., & Villar, F. (1997). Effects of electrical stimulation on wound healing in patients with diabetic ulcers. Diabetes Care, 20(3), 405–412.
Kloth, L. C., & McCulloch, J. M. (1996). Promotion of wound healing with electrical stimulation. Advances in Wound Care, 9(5), 42–45.
Carley, P. J., & Wainapel, S. F. (1985). Electrotherapy for acceleration of wound healing: Low intensity direct current. Archives of Physical Medicine and Rehabilitation, 66, 443–446.
Gurtner, G. C., & Chapman, M. A. (2016). Regenerative medicine: Charting a new course in wound healing. Advances in Wound Care, 5(7), 314–328.
Ud-Din, S., & Bayat, A. (2014). Electrical stimulation and cutaneous wound healing: A review of clinical evidence. Healthcare, 2(4), 445–467.
Hodgkin, A. L., & Huxley, A. F. (1952). A quantitative description of membrane current and its application to conduction and excitation in nerve. Journal of Physiology, 117, 500–544.
Cheng, N., Van Hoof, H., Bockx, E., et al. (1982). The effects of electric currents on ATP generation, protein synthesis, and membrane transport of rat skin. Clinical Orthopaedics and Related Research, 171, 264–272.
Decoursey, T. E. (2003). Voltage-gated proton channels and other proton transfer pathways. Physiological Reviews, 83(2), 475–579.
Ridley, A. J., Schwartz, M. A., Burridge, K., et al. (2003). Cell migration: Integrating signals from front to back. Science, 302(5651), 1704–1709.
Schwab, A. (2001). Function and spatial distribution of ion channels and transporters in cell migration. American Journal of Physiology-Renal Physiology, 280(5), F739–F748.
Chapman-Jones, D., Young, S., & Tadej, M. (2010). Assessment of wound healing following electrical stimulation with Accel-Heal. Wounds UK, 6(3), 67–71.
Wood, J. M., Evans, P. E., III, Schallreuter, K. U., et al. (1993). Pulsed low intensity direct current for healing chronic stage II and stage III decubitus ulcers. Archives of Dermatology, 129(8), 999–1009.
Gibson, J. N. A., Smith, K., & Rennie, M. J. (1988). Prevention of disuse muscle atrophy by means of electrical stimulation: Maintenance of protein synthesis. The Lancet, 2(8614), 767–770.
Erickson, M. L., Terence, M. S., Ryan, E., et al. (2017). Neuromuscular electrical stimulation training improves skeletal muscle oxidative capacity in individuals with motor-complete spinal cord injury. Muscle & Nerve, 55(5), 669–675.
Ferreira, S. B. S., Scheuts, M., & Levin, M. (2018). Modeling cell migration in a simulated bioelectrical signaling network for anatomical regeneration. In Proceedings of the ALIFE 2018 Conference, 194–201.
For Holistic Health Products and Services Go To:
Comentários