Causes, Mechanisms and Treatment of Delayed Onset of Muscle Soreness- Electrotherapy Application
- Dr Luigi Vorluni
- Feb 19
- 23 min read
Updated: May 30
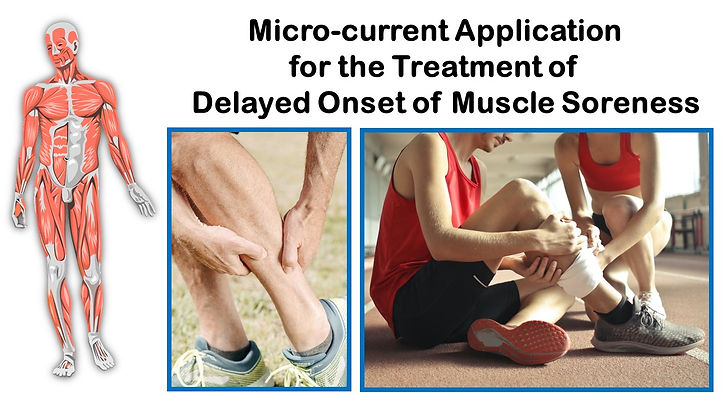
Abstract Physical exercise is critical for promoting health, as well as for enhancing fitness and sport performance. The greatest fitness and performance gains result from profound physiological responses and adaptations, which require performing high intensity and high volume training regimens. However, these types of training programmes can provoke delayed onset of muscle soreness (DOMS), which is associated with muscle tissue damage, loss of strength and coordination, as well as functional deficiency. Such muscle soreness may be experienced by both novice and elite athletes, and the severity varies from mild to very severe, which can impair function, decrease athletic performance and training capabilities. Furthermore, DOMS often lengthens the recovery time, and longer recovery times represent limitations to training at high intensity and high volume. This, in turn, impacts on the extent to which fitness and performance enhancement can be achieved. DOMS needs to be managed well, as performing physical exercise before adequate recovery is achieved, can have detrimental effects and result in more serious, debilitating and costly injuries. Even though DOMS often results from high intensity training, however, it can also be caused by unaccustomed, strenuous activities of daily living. Thus, treating DOMS is not important just for elite athletes, but also for all individuals. Electrotherapy involves the application of exogenous electrical energy to various tissues of the body, which can stimulate and enhance physiological activity, bringing about numerous therapeutic benefits. Research has revealed that electrotherapy is an efficacious intervention that can be applied to improve DOMS.
Introduction The ability to generate movement is of fundamental importance for human survival. Exercise training and sport performance involve various types of movements. Many people train to improve physical performance and for the health benefits associated with physical activity (Katch et al., 2001). The fitness and health related benefits derive from acute physiological responses and chronic adaptations to regular exercise (McArdle et al., 1986). The physical training needed to bring about health benefits is lower in volume and intensity than that necessary to bring about fitness benefits. Athletes engage in specific training programmes in order to increase performance in their respective sports (Kraemer et al., 2012). This often requires athletes to perform high intensity and high volume exercise regimens, which are necessary for enhancing physical performance (Powers & Howey, 1994). However, high workload training regimens place a great deal of stress on the body, and can lead to DOMS. Muscle soreness is an exercise-induced phenomenon that occurs several hours to days after unaccustomed or strenuous physical exercise (Talag, 1973). DOMS is classified as a type I muscle strain injury, and the symptoms include muscle stiffness and pain to palpation and/or movement, which usually peak between 24 and 72 hours after exercise (Gulik & Kimura, 1996; Safran and Seaber 1989). The aetiology of DOMS has not been fully elucidated, but it is believed that high-force muscle contractions cause micro-damage to the muscle architecture and to the sarcolemma, resulting in calcium homeostasis disruptions, immune and inflammatory responses, with accumulation of inflammatory mediators leading to the activation of nociceptors and pain development (Armstrong, 1984; Byrnes, 1986; Cleak, 1992a; Newham et al., 1982). There is evidence to suggest that eccentric contractions are more likely to result in DOMS. This because, during eccentric contractions the muscle lengthens while generating force, and the cross-bridges are separated with greater force due to disruption of the actin-myosin bonds before relaxation (Jones et al., 1986). Consequently, a greater force is developed within the active motor units, which increases the risk of injury to the myotendinous junction. In addition to causing discomfort, DOMS limit the ability to train and perform (Edgerton 1996; Garrett, 1990; MacIntire, 1995; Miles et al., 1997). Usually DOMS is a transient phenomenon, however, if not managed appropriately it may lead to more serious injuries (Edgerton et al., 1996; Smith, 1992). Causes and Mechanisms of Delayed Onset of Muscle Soreness There is evidence to suggest that DOMS development, which occurs following physical exercise, is the result of complex interactions involving various factors such as muscle damage, inflammation and calcium accumulation. Skeletal muscle tissue includes type I muscle fibres, also known as slow twitch, and type II fibres, also called fast twitch, and the content and composition of their connective tissue appears to be different. Type II muscle fibres are more susceptible to injury, as they exhibit a less robust structure compared to type I (Stauber, 1989). Thus, physical activities requiring high-force muscle contractions, may result in excessive strain of type II fibres connective tissue and cause DOMS (Hough 1902). Moreover, performing eccentric exercise can result in microscopic lesions of contractile components (Armstrong, 1984; Friden et al., 1988; Friden et al., 1984; Friden et al., 1983; Friden et al., 1981; Jones et al,. 1986; Newham et al., 1983) or even myofibrillar disruption of the z-line (Friden & Lieber, 1992), as well as more widespread disruption of the sarcomere architecture (Newham et al., 1986). This damage is more likely to occur during eccentric contractions because there is a decrease in active motor units, leading to an augmented tension per unit area (Armstrong, 1984). Besides, type II muscle fibres exhibit the narrowest and weakest z-lines, and consequently they are more prone to mechanical disruption to their structural elements. The sensation of pain arises from nociceptors activation, which are present in the musculotendinous junction, connective tissue and local vasculature. The notion of muscle damage is further supported by the high plasma levels of the enzyme creatine kinase (CK). This enzyme is present in skeletal and cardiac muscles, and is considered an indicator of membrane permeability and tissue damage (Cleak & Eston, 1992a). Following eccentric exercise, circulating CK rise dramatically above normal levels, suggesting the presence of damage to the muscle tissue and increased sarcolemma permeability (Armstrong, 1990; Brown et al., 1997; Cleak & Eston, 1992b; Newham et al., 1986; Newham et al., 1983). Even though there is a discrepancy between peak muscle soreness and peak in serum CK levels, nonetheless, micro-lesions and structural damage to the muscle tissue are important factors associated with DOMS (Clarkson & Ebbeling, 1988; Clarkson et al., 1986a; Clarkson et la., 1986b; Evans et al., 1986; Jones & Newham, 1985; Newham et al., 1986; Newham et al., 1983; Walsh et al., 2001).
Besides, repetitive eccentric exercise can lead to oedema and inflammatory cells infiltration (Evans et al., 1986; Francis, 1987; Smith, 1991), which are indicators of an inflammatory response. This occurs as a result of damage to the muscle tissue structures, and accumulation of inflammatory mediators, including bradykinin, prostaglandins and histamine at the injury site, which attract the immune cells neutrophils and monocytes (Boruker et al., 1989). The increased permeability of the local vasculature leads to an influx of exudate and oedema development (Smith, 1991). The accumulation of this protein rich fluid in the affected area, increases the osmotic pressure, activating the nociceptors. It appears that there is a relationship between peak oedema levels and peak muscle soreness (Lighfoot, 1997, Gulik & Kimura, 1997). Also, this can be exacerbated by the conversion of monocytes into macrophages, which accumulate at the injured site and produce compounds that sensitise pain receptors (Armstrong, 1984, Smith, 1991). In a healthy muscle tissue calcium is stored in the sarcoplasmic reticulum, but as a result of muscle structure and sarcolemma damage, calcium ions leak out and accumulate at the injured site (Armstrong, 1984), which can affect cellular respiration and ATP synthesis, and may also activate the enzymes proteases and phospholipases, resulting in further injury to the sarcolemma and production of the inflammatory mediators prostaglandins and leukotrienes (Armstrong, 1984 & 1990), which also can contribute to pain development.
In addition, there are other factors that can be involved in DOMS development, and these include lactic acid accumulation and muscle spasm (Clerk, 1992; Gulik and Kimura, 1996). However, lactic acid may be involved in pain occurring shortly after high-intensity exercise (Schwane et al., 1983), but it is unlikely that it plays a significant role in DOMS, because muscle soreness can last from several hours up to few days, while lactic acid levels drop considerably within one hour after the cessation of exercise (Carzola et al., 2001). A muscle spasm is associated with increased muscle tension, augmenting the intro-muscular pressure and compressing the local vasculature, leading to ischemia with accumulation of metabolites and other molecules that can cause pain. This, in turn, can increase the spasm, aggravating the ischemia (Bobber et al., 1986; de Vries, 1966). However, various studies used electromyography (EMG) to evaluate skeletal muscles activity during DOMS and the results were mixed. Some of these investigations showed no increase in EMG activity in sore muscles (Abraham, 1977; Newham et al., 1983; Talag 1973), and other investigations showed increased EMG activity, but no relationship between its magnitude and perception of soreness (Bobbert, 1986). The findings from all these studies suggest that various factors, including damage to the muscle architecture and connective tissue, inflammation and calcium accumulation at the site of injury, play a major role in DOMS development. However, the contribution of other factors to DOMS, such as lactic acid accumulation and muscle spasm cannot be ruled out. Given the numerous factors involved in DOMS and their complex interactions, there is consensus among the scientific community that muscle soreness, observed following physical exercise, is likely to be the manifestation of a multifactorial phenomenon.
Impact of Delayed Onset of Muscle Soreness on Athletic performance DOMS can be experienced by both novice and elite athletes, and the severity varies from mild, which is felt during physical activity related to daily routines, to severe pain that restricts movements (Garrett, 1990; MacIntire, 1995). The structural damage to the muscle and connective tissue, associated with DOMS, can adversely affect muscular performance, both from voluntary reduction of effort and from inherent impaired muscle capacity to generate contractile force (Armstrong, 1984). In general, the discomfort and decrease in performance are transient, and usually permanent impairment does not occur (Armstrong, 1984; Jones et al., 1986). The transient decrease in athletic performance and training capability, associated with DOMS, can be attributed to alterations in muscle function and joints mechanics (Friden et al., 1983; Harris et al., 1990; Paddon-Jones, 1997; Rowlands et al., 2001; Weber, 1994). There is evidence suggesting that during DOMS there is a decrease in muscular strength and reduced range of motion, which represent important functional limitations (Vasudevan, 1993). Moreover, the alteration in joints proprioception leads to an overestimation of force generation and impaired kinaesthetic control (Saxton, 1995), impacting on the ability to perform tasks.
Physical activity and athletic performance require the activation and coordination of different muscle groups. Depending on the task, skeletal muscles have to generate concentric, eccentric and isometric contractions in a coordinated manner, with the appropriate temporal sequencing. A number of studies revealed that parameters of strength and power during DOMS are reduced in concentric and isometric contractions, but especially in eccentric contractions (Brown et al., 1997; Clarkson & Ebbeling, 1988; Donnelly et al., 1990; Eston et al., 1996; Francis & Hoobler, 1988; Hasson et al., 1993; Nosaka & Clarkson, 1996; Paddon-Jones & Quigley, 1997; Yates, 1990). Peak torque deficit occurs 24-48 hours after exercise, and appears to be more profound and persistent during eccentric contractions (Smith, 1992). The strength levels during concentric and isometric contractions return to baseline within 5 days (Ebbeling & Clarkson, 1989), whereas it may take up to 8-10 days for strength during eccentric contractions to return to baseline (Evans et al., 1990). The altered muscle recruitment patterns and neuromuscular control, associated with DOMS, can impact on coordination, temporal sequencing and ability to perform tasks (Edgerton, 1996, Miles et al., 1997).
The severity and duration of DOMS varies among individuals, however, until full recovery from DOMS is achieved, physical performance and ability to train are compromised in both amateur and elite athletes. This because DOMS is characterised by muscle tissue damage, loss of strength and coordination, as well as functional deficiency. People striving to maintain high level of fitness, and athletes participating in sports events and competitions, need to perform high-intensity and high volume training regimens. As a consequence, many individuals tend to continue training when the muscle pain is still present, which can be detrimental (Smith, 1992). Even though DOMS is considered a sub-clinical injury, however, it needs to be managed well, and performing heavy training before adequate recovery from DOMS is achieved, can result in strain or serious and debilitating injuries (Edgerton et al., 1996, Smith, 1992).
Physical Training Regimen and Delayed Onset of Muscle Soreness When challenged with a physical task, the human body responds through a series of integrated and coordinated changes in function, which involve different physiologic systems (Abernethy et a., 1990; Ehrman et al., 2003). Physical activity is one of the most important factors for enhancing performance and improving wellness (Fagard and Tripton, 1994). The benefits of regular exercise extend into many areas of life, for people of all ages (Ehrman et al., 2003). Appropriate physical training is vital for eliciting acute physiological responses and chronic adaptations leading to the enhancement of health, fitness and performance. Scientific research into different areas of exercise physiology, has permitted the identification of the most efficient training strategies for improving physical performance. Nonetheless, enhancing the fitness of individuals and the performance capacity of athletes, is not only a matter of optimising the training regimens. It also depends on optimal balance between training and recovery, in order to prevent maladaptation to accumulated physiological and psychological stresses, induced by the training load (Meeusen et al., 2013; Soligard et al., 2016). Indeed, sport competitions and high intensity training can lead to DOMS and increased perceived fatigue (Cheung et al., 2003; Dugue, 2015; Friesenbichler et al., 2011; Ispirlidis et al., 2008).
During training, the workload should be appropriate according to the demand placed on the athlete by the specific sport discipline (Powers & Howey, 1994). A key component of exercise training, is the gradual increase of the workload in order to elicit progressively greater acute physiological responses and chronic adaptations (McArdle et al., 1986). After a period of regular training with a progressive increase of the workload, the athlete’s performance normally improves accordingly (Fox et al., 1989). Greater training workloads elicit more profound physiological responses and adaptations, which are conducive to greater fitness improvement and performance enhancement (McArdle et al., 1986). However, the training regimen should be titrated according to the athlete’s ability to respond and adapt to the exercise stimuli (Ehrman et al., 2003). The length of time between training sessions should be sufficient to allow adequate recovery (McArdle et al., 1986). It is crucial to enhance the ability to recover in a shorter period of time, because longer recovery times represent limitations to the athlete’s ability to train, which impacts on the extent of physiological adaptations and performance improvement (McArdle et al., 1986; Powers & Howey, 1994). Muscle soreness represents a major factor that influences recovery time, and performing heavy physical training or participating in sports events before the athletes recover adequately from DOMS, can have detrimental effects and increase the susceptibility to injury (Edgerton et al., 1996; Smith, 1992). Thus, it is very important to identify and implement strategies to treat DOMS.
Application of Electrotherapy to Improve Delayed Onset of Muscle Soreness Bioelectricity comprises all electrical phenomena associated with a myriad of biological processes that are essential for life. The importance of bioelectrical potentials in functions of neurones and myocytes has been long appreciated, but bioelectricity is also involved in many other physiological processes and repair mechanisms. Electrotherapy is a technique that involves the use of fine-tuned electrical impulses, which are generated by specifically engineered devices and delivered via surface electrodes placed on the skin. Electrotherapy comprises different modalities, and each modality stimulates the body in a specific way, mediating physiological changes that bring about various therapeutic benefits. Microcurrent stimulation (MCS) represents one of the electrotherapy modalities, and its distinctive characteristic is the utilisation of exogenous electrical energy in the micro-amperage range that emulates the body’s natural bioelectricity. MCS therapy can influence the physiological state, promote tissue repair and assist in the restoration of function (Kitchen & Bazin, 1996; Low & Reed, 2000). Moreover, research has revealed that MCS application is also effective in improving DOMS (Lambert et al., 2002, Naclerio et al., 2019).
Muscle soreness often occurs after competitive sport events, as well as following recreational physical activity (Clarkson & Sayers, 1999). Thus, treating DOMS is not important just for elite athletes, but also for all physically active individuals. The effectiveness of a number of interventions to improve DOMS has been investigated, including ice therapy (Yackzan et al., 1984), stretching (Buroker & Schwane, 1989), massage (Tiidus, 1997), nonsteroidal anti-inflammatory drugs (NSAIDs) and transdermal nonsteroidal anti-inflammatory agents (Barlas et al., 2000; Dudley et al., 1997; Semark et al., 1999). It appears that none of these interventions has proved to be consistently effective in improving DOMS (Cleak & Eston, 1992).
There is evidence suggesting that MCS is an efficacious tool that can be applied to improve DOMS. A double blind, placebo controlled study evaluated the effect of MCS therapy on DOMS (Lambert et al., 2002). Eccentric exercise was used to provoke DOMS in the flexor muscles of the elbow. In all participants, the elbow flexor muscles were assessed intermittently for up to 7 days following exercise, for muscle soreness, function, inflammation, and passive shortening. The results showed that MCS therapy improved different clinical manifestations of tissue damage, including decreased serum CK levels, reduced muscle shortening and maintenance of maximum force production of the elbow flexor muscles. Besides, Naclerio and co-workers (2019), conducted a double blind, placebo controlled study, to evaluate the effects of resistance training in combination with either MCS or sham treatment on DOMS. A specific exercise protocol was implemented to induce DOMS. Participants followed an identical resistance training routine, consisting of 3 sessions per week, for a total of 8 weeks, and received either a 3-hour daily MCS therapy or sham therapy, immediately post workout or during the morning on non-training days. All participants had at least 2 years of resistance training experience. The results revealed that MCS application attenuated DOMS, which was assessed over a period of 12-h to 48-h. Curtis and colleagues (2010) compared the effects of MCS therapy versus sham therapy on DOMS, which was induced by implementing an eccentric contractions exercise protocol. The individuals who took part in the study included young males and females. Muscle soreness was assessed at baseline and at 24, 48 and 72 hours post-exercise. The authors reported that MCS application provided significant protection from DOMS at all time points tested. The improvements of DOMS, derived from MCS application can be attributed to its ability to re-establish normal endogenous current flow to the injured tissue (Becker, 1961; Mercola & Kirsch, 1995), augment ATP and protein synthesis, and increase transport of metabolites across the plasma membrane (Cheng et al., 1982), as well as accelerating the repair processes (Bayat et al., 2006; Lessiani et al., 2014). These physiological changes help re-establish homeostasis in the traumatised tissue, creating an optimal environment that promotes healing. Besides, the ability of MCS to ameliorate the symptoms of muscle soreness, are derived from its electroanalgesic and anti-inflammatory properties (Kirsch & Lerner, 1990; Lee et al., 2011).
Transcutaneous electrical nerve stimulation (TENS) represents another electrotherapy modality that is efficacious in alleviating pain. Graig and associate (1992), evaluated the effect of TENS and other standard treatments for improving DOMS. The participants included young female individuals, and DOMS was induced in the non-dominant elbow flexor muscle group by performing repeated eccentric contractions. The results showed that the improvement of DOMS, mediated by TENS application, was significantly greater compared to sham TENS. The role of TENS as an electroanalgesic agent is well established. Research has revealed that this technique is effective in treating different types of pain (Bilgili et al., 2016; Bohm, 1978; Cheing et al., 1999; Fishbain et al., 1996; Mannheimer & Carlsson, 1979; Melzak et al., 1983). Depending on the stimulation parameters settings, TENS administration can mediate analgesia through segmental, extrasegmental and peripheral mechanisms (Chung et al., 1984a, b; Duranti et al., 1988; Garrison and Foreman, 1996; Ignelzi & Nyquist, 1976; Le bars et al., 1979; Sjolund, 1985; Sjolund, et al., 1977; Wagman, 1969; Woolf et al., 1988; Woolf et al., 1980).
Considerations and Conclusion Physical exercise promotes health, and ameliorates sport performance and fitness. Performing high intensity and high volume training regimens are associated with profound physiological responses and adaptations that bring about the greatest fitness and performance gains. However, these types of training programmes can result in DOMS, which may be experienced by both novice and elite athletes. The symptoms of DOMS include loss of strength and coordination, as well as functional deficiency, which can impact on the ability to perform daily tasks, as well as during sport events. Moreover, DOMS is associated with longer recovery time, which impacts on the ability to perform high intensity and high volume training, affecting the extent to which fitness and performance enhancement can be achieved. Although DOMS is often caused by high intensity training, however, it can also result from unaccustomed, strenuous activities of daily living, and thus, treating muscle soreness is very important for all individuals. Electrotherapy is a safe and effective technique that stimulates physiology and the body’s natural healing ability, promoting health and wellness. Electrotherapy represents an efficacious intervention that can be applied to improve DOMS. The efficacy of electrotherapy is not limited to DOMS treatment, as this cutting-edge energy medicine approach is very versatile, and can be applied in a wide range of sport, fitness and clinical settings.
References
Abernethy PJ, Thayer R, Taylor AW. Acute and chronic responses of skeletal muscle to endurance and sprint exercise: a review. Sports Medicine; 1990, 10:365–389.
Abraham WM. Factors in delayed muscle soreness. Med Sci Sport Exerc; 1977, 9 (1): 11-20.
Armstrong RB. Mechanisms of exercise-induced delayed onset: a brief review. Med Sci Sports Exerc, 1984, 16 (6): 529-38.
Armstrong R. Initial events in exercise-induced muscular injury. Med Sci Sports Exerc; 1990, 22 (4): 429-35
Barlas, P., J. A. Craig, J. Robinson, D. M. et al. Managing delayed-onset muscle soreness: Lack of effect of selected oral systemic analgesics. Arch. Phys. Med. Rehabil. 2000, 81:966–972.
Bayat M, Asgari-Moghadam Z, Maurofi M et al. Experimental wound healing using microamperage electrical stimulation in rabbits. J Rehab Res Dev, Mar-Apr; 2006 43(2):219-26.
Becker RO. The bio electric factors in amphibian limb regeneration. J Bone Joint Surg;1961, 43A:643-656.
Bilgili A, Cakir R, Dogan SK, et al. The effectiveness of transcutaneous electrical nerve stimulation in the management of patients with complex regional pain syndrome: A randomized, double-blinded, placebo-controlled prospective study. J Back and Musculoskeletal Rehabil. 2016, Nov 21;29(4):661-671
Bohm E. Transcutaneous electrical nerve stimulation in chronic pain after peripheral nerve injury. Acta Neurochir (Wien), 1978, 40(3-4):277-283. doi:10.1007/BF01774752
Buroker, K. J. and Schwane J. A. Does post-exercise static stretching alleviate exercise-induced delayed muscle soreness? Physician Sportsmed.1989, 17:65–83.
Brown SJ, Child RB, Day SH, et al. Indices of skeletal muscle damage and connective tissue breakdown following eccentric muscle contractions. Eur J Appl Physiol Occup Physiol; 1997, 75 (4): 369-74
Bobbert MF, Hollander AP, Huijing PA. Factors in delayed onset muscular soreness of man. Med Sci Sports Exerc; 1986, 18 (1): 75-81
Buroker KC, Schwane JA. Does postexercise stretching alleviate delayed muscle soreness? Phys Sports Med; 1989, 17 (6): 65-83
Byrnes WC, Clarkson PM. Delayed onset muscle soreness and training. Clin Sports Med; 1986, 5 (3): 605-14.
Cazorla G, Petibois C, Bosquet L, et al. (2001) Lactate et exercice: mythes et realites. Rev Sci Tech Activ Phys Sport (Grenoble); 22 (54): 63-76.
Cheng N, Van Hoff H, Bockx E, et al. (1982) The effect of electric currents on ATP generation protein synthesis, and membrane transport in rat skin. Clin, Orthop. 171:264-72.
Cheing GL and Hui-Chan CW. (1999) Transcutaneous electrical nerve stimulation: nonparallel antinociceptive effects on chronic clinical pain and acute experimental pain. Arch Phys Med Rehabil. Mar;80(3):305-12. doi: 10.1016/s0003-9993(99)90142-9
Cheung, K., Hume, P., and Maxwell, L. (2003). Delayed onset muscle soreness: treatment strategies and performance factors. Sports Med. 33, 145–164. doi: 10.2165/00007256-200333020-00005
Clarkson PM, Ebbeling C. (1988) Investigation of serum creatine kinase variability after muscle damaging exercise. Clin Sci; 75: 257-61
Clarkson PM, Byrnes WC, McCormick KM, et al. (1986) Muscle soreness and serum creatine kinase activity following isometric, eccentric and concentric exercise. Int J Sports Med;7:152-5,a
Clarkson PM, Apple FS, Byrnes WC, et al. (1986) Creatine kinase isoforms following isometic exercise. Muscle Nerve; 10 (1): 41-4,b
Clarkson, P. M., and S. P. Sayers. (1999) Etiology of exercise-induced muscle damage. Can. J. Appl. Physiol. 24:234–48.
Cleak MJ, Eston RG, (1992a) Delayed onset muscle soreness: mechanisms and management. J Sports Sci; 10 (4): 325-41.
Cleak MJ, Eston RG, (1992b) Muscle soreness, swelling, stiffness and strength loss after intense eccentric exercise. Br J Sports Med; 26 (4): 267-72
Chung, JM, Fang, ZR, Hori, Y, et al. (1984) Prolonged inhibition of primate spinothalamic tract cells by peripheral nerve stimulation. Pain, 19: 259–275
Chung, JM, Lee, KH, Hori, et al. (1984b) Factors influencing peripheral nerve stimulation produced inhibition of primate spinothalamic tract cells. Pain 19: 277–293
Curtis D, Fallows S, Morris M, McMakin C (2010) The efficacy of frequency specific microcurrent therapy on delayed onset muscle soreness. J Bodyw Mov Ther 14:272–279.
de Vries HA. (1996) Quantitative EMG investigation of the spasm theory of muscle pain. Am J Phys Med; 45: 119-34
Donnelly AW, Maughan RJ, Whiting PH. (1990) Effects of ibuprofen on exercise-induced muscle soreness and indices of muscle damage. Br J Sports Med; 24 (3): 191-5
Dugue, B. M. (2015). An attempt to improve Ferreira-Junior model concerning the anti-inflammatory action of whole-body cryotherapy after exercise induced muscular damage (EIMD). Front Physiol. 6:35. doi: 10.3389/fphys.2015.00035.
Dudley, G. A., J. Czerkawski, A. Meinrod, G. et al. (1997) Efficacy of naproxen sodium for exerciseinduced dysfunction muscle injury and soreness. Clin. J. Sport. Med. 7:3–10.
Duranti, R, Pantaleo, T, Bellini, F (1988) Increase in muscular pain threshold following low frequency-high intensity peripheral conditioning stimulation in humans. Brain Research 452: 66–72
Ebbeling CB, Clarkson PM. (1989) Exercise-induced muscle damage and adaptation. Sports Med; 7 (4): 207-34
Edgerton VR, Wolf SL, Levendowski DJ, et al. (1996) Theoretical basis for patterning EMG to assess muscle function. Med Sci Sports Exerc; 28 (6): 744-51
Ehrman JK, Gordon PM, Visich PS, et al., Clinical exercise physiology. 2003, Human Kinetics Publishes, Inc.
Eston RG, Finney S, Baker S, et al. (1996) Muscle tenderness and peak torque changes after downhill running following a prior bout of isokinetic eccentric exercise. J Sports Sci (London); 14 (4): 291-9
Evans WJ, Meredit CN, Cannon JG, et al. (1986) Metabolic changes following eccentric exercise in trained and untrained men. J Appl Physiol; 61 (5): 1864-8
Evans DT, Smith LL, Chenier TC, et al. (1990) Changes in peak torque, limb volume and delayed onset muscle soreness following repetitive eccentric contractions. Int J Sports Med; 11: 403
Fagard RH, Tipton CM. Physical activity, fitness, and hypertension. In: Bouchard C, Shephard RJ, Stephens T, editors. Physical activity, fitness, and health: international proceedings and consensus statement. Champaign, IL: Human Kinetics, 1994:633–655.
Fishbain DA, Chabal C, Abbott A, et al. (1996) Transcutaneous electrical nerve stimulation (TENS) treatment outcome in longterm users. Clin J Pain, 12:201–214
Fox EL, Bowers RW, Foss ML. Physiological basis of physical education and athletics. 1989, Wm C Brown publishers.
Francis KT, Hoobler T. (1987) Effects of aspirin on delayed muscle soreness. J Sports Med Phys Fitness; 27 (3): 333-7
Francis K, Hoobler T. (1988) Delayed onset muscle soreness and decreased isokinetic strength. J Appl Sport Sci Res; 2 (2): 20-3
Friesenbichler, B., Stirling, L. M., Federolf, P., et al., (2011). Tissue vibration in prolonged running. J. Biomech. 44, 116–120. doi: 10.1016/j.jbiomech.2010.08.034
Friden J, Seger J, Ekblom B. (1988) Sublethal muscle fibre injuries after high-tension anaerobic exercise. Eur J Appl Physiol; 57: 360-8
Friden J, Kjorell U, Thornell LE. (1984) Delayed muscle soreness and cytoskeletal alterations: an immunocytological study in man. Int J Sports Med; 5: 15-8
Friden J, Sjostrom M, Ekblom B. (1983) Myofibrillar damage following intense eccentric exercise in man. Int J Sports Med; 4: 170-6
Friden J, Sjostrom M, Ekblom B(1981) A morphological study of delayed onset muscle soreness. Experentia; 37: 506-7
Friden J, Lieber RL. (1992) Structural and mechanical basis of exercise induced muscle injury. Med Sci Sports Exerc; 24 (5): 521-30
Garrett JWE. (1990) Muscle strain injuries: clinical and basic aspects. Med Sci Sports Exerc; 22 (4): 436-43.
Graig RD, Perrin D. (1992) Effect of Transcutaneous Electrical Nerve Stimulation, Cold, and a Combination Treatment on Pain, Decreased Range of Motion, and Strength Loss Associated with Delayed Onset Muscle Soreness. Journal of Athletic Training, 27, 200-206.
Garrison, D, Foreman, R (1996) Effects of transcutaneous electrical nerve stimulation (TENS) on spontaneous and noxiously evoked dorsal horn cell activity in cats with transected spinal cords. Neuroscience Letters 216: 125–128
Gulick DT, Kimura IF. (1996) Delayed onset muscle soreness: what is it and how do we treat it? J Sport Rehab; 5: 234-43
Harris C, Wilcox A, Smith G, et al. (1990) The effect of delayed onset muscle soreness (DOMS) on running kinematics. Med Sci Sport Exerc; 22 (2): S34
Hasson SM, Daniels JC, Divine JG, et al. Effect of ibuprofen use on muscle soreness, damage, and performance: a preliminary investigation. Med Sci Sports Exerc 1993; 25 (1): 9-17
Hough T. Ergographic studies in muscular soreness. Am J Physiol 1902; 7: 76-92
Ignelzi, RJ, Nyquist, JK (1976) Direct effect of electrical stimulation on peripheral nerve evoked activity: implications in pain relief. Journal of Neurosurgery 45: 159–165
Ispirlidis, I., Fatouros, I. G., Jamurtas, A. Z., et al. (2008). Time-course of changes in inflammatory and performance responses following a soccer game. Clin. J. Sport Med. 18, 423–431. doi: 10.1097/JSM.0b013e3181818e0b
Jones DA, Newham DJ, Round JM, et al. Experimental human muscle damage: morphological changes in relation to other indices of damage. J Physiol 1986; 375: 435-48.
Jones DA, Newham DJ. The effect of training on human muscle pain and damage. J Physiol 1985; 365: 76
Katch VL, McArdle WD, Katch FI. Essentials of exercise physiology. 2011, Lippincott Williams & Wilkins, a Wolters Kluwer business
Kirsch D, Lerner F. Innovations In pain management: a practical guide for clinicians. In: Weiner RL (ed) Electro medicine 1990; Deutsche Press; 23:1-29.
Kitchen S, Bazin S, eds. Clayton’s Electrotherapy, 10th edn. Philadelphia: WB Saunders, 1996.
Kraemer WJ, Flack SJ, Deschenes MR. Exercise Physiology. Integrating theory and application. 2012 Lippincott Williams & Wilkins, a Wolters Kluwer business
Lambert MI, Marcus P, Burgess T, et al. Electro-membrane microcurrent therapy reduces signs and symptoms of muscle damage. Med Sci Sports Exerc. 2002 Apr;34(4):602-7.
Lee JW, Yon SW, and Kim TH et al. The effects of microcurrent on inflammatory reactions induced by ultraviolet light. J Phys Ther Sci. 2011, 23: 693-696
Lightfoot JT, Char D, McDermott J, et al. Immediate postexercise massage does not attenuate delayed onset muscle soreness. J Strength Cond Research 1997; 11 (2): 119-24
Low J, Reed A. Electrotherapy Explained: Principles and Practice, 3rd edn. Oxford: Butterworth Heinemann, 2000.
Mannheimer, C, Carlsson, C. (1979) The analgesic effect of transcutaneous electrical nerve stimulation (TNS) in patients with rheumatoid arthritis. A comparative study of different pulse patterns. Pain, 6: 329–334
McArdle WD, Katch I, Katch L. Exercise Physiology, Energy Nutrition and Human Performance. 1986, Lea and Fabiger.
MacIntyre DL, Reid WD, McKenzie DC. Delayed muscle sore ness: the inflammatory response to muscle injury and its clinical implications. Sports Med 1995; 20 (1): 24-40.
Meeusen, R., Duclos, M., Foster, C., et al. (2013). Prevention, diagnosis, and treatment of the overtraining syndrome: joint consensus statement of the European College of Sport Science and the American College of Sports Medicine. Med. Sci. Sports Exerc. 45, 186–205. doi: 10.1249/MSS.0b013e318279a10a
Melzak R, Vetere P, Finch L. (1983) Transcutaneous electrical nerve stimulation for low back pain. A comparison of TENS and massage for pain and range of motion. Phys Ther. Apr;63(4):489-93. doi: 10.1093/ptj/63.4.489
Le Bars D, Dickenson AH, Besson JM, (1979) Diffuse noxious inhibitory controls (DNIC): 2. Lack of effect on non-convergent neurons, supraspinal involvement and theoretical implications. Pain, 6:305-327
Lessiani G, Galati V, Franzone G, et al. Efficacy of Modulated Microcurrent Stimulation in Pressure Ulcers Treatment: A Monocentric, Prospective, Double-Blind, Randomized Study. J Nov Physiother 2014, 4:4.
Miles MP, Ives JC, Vincent KR. Neuromuscular control following maximal eccentric exercise. Eur J Appl Physiol 1997; 76: 368-74
Mercola JM and Kirsch DL. The Basis for Micro Current Electrical Therapy in Conventional Medical Practice. Journal of Advancement in Medicine, Volume 8, Number 2, Summer 1995
Naclerio F, Seijo M, Karsten B, et al. Effectiveness of combining microcurrent with resistance training in trained males. European Journal of Applied Physiology (2019) 119:2641–2653
Newham DJ, Jones DA, Edwards RHT. Plasma creatine kinase changes after eccentric and concentric contractions. Muscle Nerve 1986; 9: 59-63
Newham DJ, Mills KR, Edwards RHT. Large delayed plasma creatine kinase changes after stepping exercise. Muscle Nerve 1983; 6: 380-5.
Newham DJ, Mills KR, Quigley R, et al. Muscle pain and tenderness after exercise. Aust J Sports Med Exerc Sci 1982; 14: 129-31.
Nosaka K, Clarkson PM. Changes in indicators of inflammation after eccentric exercise of the elbow. Med Sci Sports Exerc, 1996; 28 (8): 953-61
Paddon-Jones DJ, Quigley BM. Effect of cryotherapy on muscle soreness and strength following eccentric exercise. Int J Sports Med 1997; 18: 588-93
Powers SK, Howley ET. Exercise Physiology, Theory and Application to Fitness and Performance. 1994, Scott Spoolman editor.
Rowlands AV, Eston RG, Tilzey C. Effect of stride length manipulation on symptoms of exercise-induced muscle damage and the repeated bout effect. J Sports Sci 2001; 19 (5): 333-40
Safran MR, Seaber AV, Garrett JWE. Warm-up and muscular injury prevention, an update. Sports Med 1989; 8 (4): 239-49
Semark, A., T. D. Noakes, A. St Clair Gibson et al., The effect of a prophylactic dose of flurbiprofen on muscle damage, soreness and sprinting performance in trained subjects. J. Sports Sci. 17:197–203, 1999.
Saxton JM, Clarkson PM, James R, et al. Neuromuscular dysfunction following eccentric exercise. Med Sci Sports Ex erc 1995; 27 (8): 1185-93
Schwane JA, Hatrous BG, Johnson SR, et al. Is lactic acid related to delayed-onset muscle soreness? Phys Sports Med. 1983; 11 (3): 124-7, 130-1.
Sjolund, B. (1985) Peripheral nerve stimulation suppression of C-fiber-evoked flexion reflex in rats. Part 1: Parameters of continuous stimulation. Journal of Neurosurgery 63: 612–616
Sjolund, B, Terenius, L, Eriksson, M (1977) Increased cerebrospinal fluid levels of endorphins after electro-acupuncture. Acta Physiologica Scandinavica 100: 382–384
Smith LL. Causes of delayed onset muscle soreness and the impact on athletic performance: a review. J Appl Sport Sci Res 1992; 6 (3): 135-41
Smith LL. Acute inflammation: the underlying mechanism in delayed onset muscle soreness? Med Sci Sports Exerc 1991; 23 (5): 542-51
Stauber WT. Eccentric action of muscles: physiology, injury and adaptation. In: Pandolf KP, editor. Exercise and sport science reviews. Baltimore (MD): Williams and Wilkins, 1989: 157-86
Soligard, T., Schwellnus, M., Alonso, et al. (2016). How much is too much? (Part 1) International Olympic Committee consensus statement on load in sport and risk of injury. Br. J. Sports Med. 50, 1030–1041. doi: 10.1136/bjsports-2016-096581
Talag T. Residual muscle soreness as influenced by concentric, eccentric, and static contractions. Res Q 1973; 44: 458-69.
Tiidus, P. M. Manual massage and recovery of muscle function following exercise: a literature review. J. Orthop. Sports Phys. Ther. 25:107–112, 1997.
Vasudevan SV. Impairment, disability and functional capacity assessment. In: Turk DC, Melzack RM, editors. Handbook of pain assessment. New York: The Guilford Press, 1993: 100-1
Wagman IH, Price DD. (1969) Responses of dorsal horn cells of M. mulatta to cutaneous and sural nerve A and C fiber stimuli. J Neurophysiol, 32:803-817
. Walsh B, Tonkonogi M, Malm C, et al. Effect of eccentric exercise on muscle oxidative metabolism in humans. Med Sci Sports Exerc 2001; 33 (3): 436-41
. Weber MD, Servedio FJ, Woodall WR. The effects of three Electromodalities on delayed onset muscle soreness. J Sports Phys Ther 1994; 20 (5): 236-42
. Woolf, C, Thompson, S, King, A (1988) Prolonged primary afferent induced alterations in dorsal horn neurones, an intracellular analysis in vivo and in vitro. Journal of Physiology 83: 255–266
. Woolf, CJ, Mitchell, D, Barrett, GD (1980) Antinociceptive effect of peripheral segmental electrical stimulation in the rat. Pain 8: 237–252
. Yackzan I, Adams C, Francis KT. The effects of ice massage on delayed muscle soreness. Am. J. Sports Med. 12:159–165, 1984.
. Yates JW, Armbruster WJ. Concentric and eccentric strength loss and recovery following exercise induced muscle soreness. Int J Sports Med 1990; 11: 403
Comments